Mixing of an Upward Discharging Inclined Dense jet
Introduction
Wastewater effluents with a density higher than that of the environment are often discharged into coastal waters in the form of submerged dense (negatively buoyant) jets. Examples include brine discharge from desalination plants, cooled water from liquefied natural gas plants, and gypsum (hydrated calcium sulphate) waste from fertilizer factories.
In recent years seawater desalination has been adopted or planned in coastal cities (e.g., Singapore, Adelaide, Hong Kong) as a key or alternative source of water supply to address challenges of water security. As a by-product of desalination, concentrated brine solution has to be returned to a neighbouring water body. The concentrated brine effluent is typically discharged from a submarine outfall as inclined high-velocity dense jets to maximize the initial dilution. As the brine discharge is denser than the surrounding seawater, the jet eventually falls back onto the sea floor where it continues to spread horizontally as bottom gravity current.
The salinity of the brine effluent is around 50 - 70 ppt and the initial density difference (Δρ/Δρa) is about 0.010 ~ 0.035. High level of salinity can have a significantly impact on the sea bed ecology and marine biota such as the development of species, survival of larva and breeding and reproductive traits. It is necessary to achieve effective mixing of the brine discharge to minimize environmental impact and to prevent the recirculation of the concentrated brine into the seawater intake. The prediction of the mixing of dense jets is necessary for brine effluent outfall design and environmental impact assessment.
Key features for an upward discharging inclined dense jet
Consider the release of a negatively buoyant (dense) jet from the sea bed (Fig. 1). The jet momentum is opposite to direction of buoyancy which results in buoyant instability. The inclined dense jet discharge can be characterized by the jet discharge volume flux, kinematic momentum flux and buoyancy flux. The key jet characteristics include the maximum height of rise; initial dilution; bottom impingement and impact dilution.
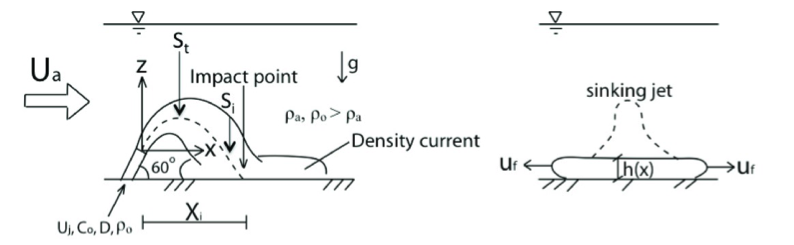
Figure 1. General characteristics of a dense jet impinging on a bottom boundary.
The dense jet is momentum-dominated before terminal rise. Detrainment is a unique feature in dense jets not found in conventional positively buoyant jet. For the laboratory experiment, it is observed that there is flow reversal before terminal rise and significant detrainment and peeling off at very small distance from source (see videos). The detrainment process is complex and not accounted for in integral jet models. VISJET correctly predicts the variation of jet property with jet angle (Fig. 2), although the jet trajectory and jet dilution are under-predicted by about 10-15% and 30% respectively.
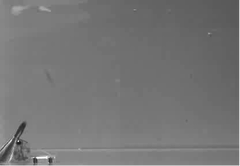
Laboratory experiment |
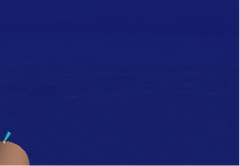
VISJET simulation |
Videos above: A 60° upward discharging dense jet in stagnant water with jet diameter D=5mm and Jet densimetric Froude number Fr = Uo/((Δρo/ρa)gD)1/2 = 16.
Figure 2. Predicted and measured minimum dilution at terminal rise St of inclined dense jets (Fr > 20) as a function of jet discharge angle.
Mixing of dense jet in a cross current
An ambient current is usually present in the coastal environment, predicting the mixing and transport of the dense effluent in moving water is essential for environmental risk assessment. After reaching its terminal height of rise, the dense jet falls and impacts on the bottom boundary; the jet flow transits into a horizontal density current with additional mixing beyond the impingement point (Fig. 1). This buoyant spreading regime is characterized by a gradual die-off of jet-induced turbulence because of the stable density stratification set up in the spreading layer; it marks the end of the active mixing zone, and is termed herein the intermediate field beyond which pollutant tracer is passively transported by ocean currents.
For the laboratory experiments, it can be observed that the crossflow Froude number F = UrFr = Ua/((Δρo/ρa)gD)1/2 delineates the behaviour of the spreading current of impinging dense jets into the three regimes. As F increases, tracer concentration (dilution) becomes less and less uniform across the spreading layer. This is most evident when the dense jet has completely bent over before its descent (F > 0.8), which results in the bifurcation of the intermediate field.
The mixing and transport in the intermediate field can be predicted using the DESA method (Choi and Lee 2007, Choi et al. 2016). Fig. 3 shows the trajectory of a dense jet predicted by the nearfield model JETLAG and the corresponding sources and sinks in the far-field model with a non-uniform vertical grid for resolving the bottom spreading layer using DESA.
Figure 3. Computed plume and source (+) / sink (o) terms in the far field model for a 60° inclined dense jet discharging in coflow direction.
The intermediate field of 60° dense jets discharged into a coflowing current has been studied experimentally and numerically (Choi et al. 2016). Fig. 4 shows the observed and predicted buoyant spread for the crossflow-dominated cases (F > 0.8); both experiments and theory show the concentration Field has a distinct bimodal structure. The predicted features of the quasi-steady buoyant spread are well-supported by the experimental data. A close match between the predicted and measured impact point dilution is also achieved for both coflow and counterflow dense jet (Fig. 5).
Figure 4. Observed and predicted lateral spread of 60° dense jets (F > 0.8).
Figure 5. Comparison of predicted minimum dilution (hollow symbol) for 60° inclined dense jet in coflow and counterflow with data (solid symbol) of Roberts and Toms (1987).
Key Publications
- Choi, K.W., Lai, C.C.K., and Lee, J.H.W., (2016), "Mixing in the Intermediate Field of Dense Jets in Cross Currents", Journal of Hydraulic Engineering, ASCE, Vol. 142, No. 1, DOI: 10.1061/(ASCE)HY.1943-7900.0001060.
- Lai, C.C.K. and Lee, J.H.W. (2014), "Initial mixing of inclined dense jet in perpendicular crossflow", Environmental Fluid Mechanics, Vol. 14, pp. 25-49.
- Lai, C.C.K. and Lee, J.H.W. (2012), "Mixing of Inclined Dense Jets in Stationary Ambient", Journal of Hydro-environment Research, Vol. 6(1), pp. 9-28.
- Choi, K.W. and Lee, J.H.W., (2007), "Distributed entrainment sink approach for modelling mixing and transport in the intermediate field", Journal of Hydraulic Engineering, ASCE, Vol. 133, No. 7, pp. 804-815.